Test Data AC7-02: Difference between revisions
No edit summary |
m (Remove links to lib section) |
||
(35 intermediate revisions by 2 users not shown) | |||
Line 8: | Line 8: | ||
=Test Data= | =Test Data= | ||
==Overview of Tests== | ==Overview of Tests== | ||
==Description of | |||
PIV measurements were performed at six different planes within the lung replica (see [[#Figure6|figure 6]]). | |||
The first three planes were located within the inlet tube with the aim to determine the exact inlet conditions. | |||
Plane IV corresponds to the central plane of the oral cavity and the pharynx. | |||
Note that this plane is tilted by <math>2^o</math> from the exact vertical orientation. | |||
The larynx and the upper trachea were imaged by plane V, and plane VI covers the main bifurcation and bronchi. | |||
Both planes are vertical. | |||
For all of the measurement stations, the mean velocity and turbulent kinetic energy fields from the in-plane velocity components are determined (see section [[Evaluation AC7-02|Evaluation]] and equations 8-11). | |||
<div id="Figure6"></div> | |||
[[File:Brno_PIV_Figure6.png| center | thumb |500px|'''Figure 6''': Locations of the PIV measurement planes I-VI. | |||
(a) Measurement planes near the inlet: I-xy plane, II-xz plane, III-yz plane. | |||
(b) Measurement planes in mouth-throat (IV-xy plane tilted by <math>2^o</math> from the exact vertical orientation), trachea (V-xy plane) and main bifurcation and bronchi (VI-yz plane).]] | |||
==Description of experiment== | |||
The lung model was placed in an open liquid tank with a piston diaphragm pump attached to a linear actuator to achieve a quasi-stationary inspiratory flow. | |||
The stroke of the piston followed a cyclic triangular function with an adjustable falling constant slope and thus constant velocity to match different flow rates during inspiration. | |||
The employed working fluid is a mixture of water/glycerine (43:57 mass ratio, <math> \rho=1150kg/m^3, \, \nu=8.4\cdot 10^{-6} m^2/s </math> at <math>20^oC </math>), | |||
which exactly matches the refractive index of the silicone model. | |||
Neutrally buoyant polyamide particles (<math >d_p = 50\mu m </math>) were used as tracer particles (Stokes number Stk ≈ 0.002). | |||
Particle images were recorded using a CCD camera (pco.1600, PCO) with a resolution of 1600px x 1200px in double-frame mode. | |||
A total number of 688 image pairs were acquired for each measurement. | |||
Illumination was provided by a double-pulse Nd:YAG laser (<math > \lambda </math> = 532nm, MinilitePIV, Continuum, 10 mJ per pulse). | |||
Images were evaluated by DaVis 8 (LaVision). | |||
The final grid size is 32 x 32px (planes I-III) and 16 x 16px (planes IV-VI), respectively, both with 50% overlap. | |||
==Boundary conditions== | ==Boundary conditions== | ||
The mouth inlet and distal outlets of the lung model are open to the surrounding liquid. | |||
Thus liquid can enter and leave the model without any additional resistances or compliances. | |||
The local pressure at the mouth inlet corresponds to the barometric pressure plus the pressure of the water column above the model which is approximately 10cm. | |||
Accordingly, the pressure at the distal outlet increases by the additional water column which corresponds to the total model height of about 50cm. During inspiration, liquid is sucked into the model from the tank. | |||
The distance between tank's walls and the inlet of the model is about 15cm. | |||
The specific inlet conditions found in the experiment are presented in [[#Figure7|figure 7]]. | |||
<div id="Figure7"></div> | |||
[[File:PIV_inlet2.png|center|thumb|500px|'''Figure 7''': Inlet flow conditions of the experiments as determined by the PIV measurements. Illustrated is the mean in-plane velocity magnitude at the planes I (a), II (b) and III (c) for a flowrate of 60 L/min. | |||
The velocity along the profile lines depicted in (a) and (b) are plotted in (d) and(e), respectively (PIV (o) and LES1 (-)).]] | |||
==Measurement errors== | ==Measurement errors== | ||
For the experiments, the Reynolds similarity is calculated based on the properties of air at <math> 25^oC </math> and atmospheric pressure, which results in a flow rate of <math> Q_{w/g} </math> = 31.75 L/min. | |||
This values has been used to program the linear actuator in order to provide the necessary main flow. | |||
At <math> Q_{w/g} </math> = 31.75 L/min, the experimental setup reaches its limit of the planned operation and a discrepancy between the targeted and actual occurring flow rate is observed. | |||
Thus, a maximum flowrate of <math> Q_{w/g} </math> = 28.56 L/min is achieved. | |||
This lowers the theoretical maximum Reynolds number from 4921 to 4286 but the flow still stays well within the turbulent regime. | |||
The reduction of the maximum Reynolds number occurs due to a leakage flow between the upper and lower part of the model. | |||
Consequently, the achieved flowrate within the main carina area is about 10% lower than in the upper part of the model. | |||
To account for this, we estimated the real flowrate based on the PIV data and recalculated the theoretical bulk velocity based on this estimate. | |||
This bulk velocity is then used as the correct scaling parameter for the results shown below. | |||
The measurement uncertainty of the displacement calculation for PIV is typically given as 0.1px ([[Best Practice Advice AC7-02#Adrian2011|Adrian and Westerweel, 2011]]). | |||
With an adjusted mean displacement of around 8px this leads to a relative error of around 1.25% in the displacement calculation. | |||
Based on the proposed methods of [[Best Practice Advice AC7-02#Sciacchitano2016|Sciacchitano and Wieneke (2016)]] the statistical uncertainty of the derived mean velocity is around 0.05% and the statistical uncertainty of the turbulent kinetic energy estimation is around 3. | |||
==Measured data== | |||
The measured in-plane velocity and turbulent kinetic energy profiles at stations B-D and G,H,J (shown in [[Evaluation AC7-02#Figure23|figure 23]] and [[Evaluation AC7-02#Figure24|24]]), can be found in file [[Media:PIV_profiles.xlsx|PIV_profiles.xlsx]]. | |||
<br/> | <br/> | ||
---- | ---- | ||
{{ACContribs | {{ACContribs | ||
|authors=P. Koullapis<sup>a</sup> | |authors=P. Koullapis<sup>a</sup>, J. Muela<sup>b</sup>, O. Lehmkuhl<sup>c</sup>, F. Lizal<sup>d</sup>, J. Jedelsky<sup>d</sup>, M. Jicha<sup>d</sup>, T. Janke<sup>e</sup>, K. Bauer<sup>e</sup>, M. Sommerfeld<sup>f</sup>, S. C. Kassinos<sup>a</sup> | ||
|organisation=<br><sup>a</sup>Department of Mechanical and Manufacturing Engineering, University of Cyprus, Nicosia, Cyprus<br> | |organisation=<br><sup>a</sup>Department of Mechanical and Manufacturing Engineering, University of Cyprus, Nicosia, Cyprus<br> | ||
<sup>b</sup>Heat and Mass Transfer Technological Centre, Universitat Politècnica de Catalunya, Terrassa, Spain<br> | |||
<sup>c</sup>Barcelona Supercomputing center, Barcelona, Spain <br> | |||
<sup>d</sup>Faculty of Mechanical Engineering, Brno University of Technology, Brno, Czech Republic<br> | |||
<sup>e</sup>Institute of Mechanics and Fluid Dynamics, TU Bergakademie Freiberg, Freiberg, Germany<br> | |||
<sup>f</sup>Institute Process Engineering, Otto von Guericke University, Halle (Saale), Germany <br> | |||
}} | }} | ||
{{ACHeader | {{ACHeader | ||
Line 21: | Line 81: | ||
|number=02 | |number=02 | ||
}} | }} | ||
© copyright ERCOFTAC 2020 |
Latest revision as of 14:33, 27 October 2022
Airflow in the human upper airways
Application Challenge AC7-02 © copyright ERCOFTAC 2020
Test Data
Overview of Tests
PIV measurements were performed at six different planes within the lung replica (see figure 6). The first three planes were located within the inlet tube with the aim to determine the exact inlet conditions. Plane IV corresponds to the central plane of the oral cavity and the pharynx. Note that this plane is tilted by from the exact vertical orientation. The larynx and the upper trachea were imaged by plane V, and plane VI covers the main bifurcation and bronchi. Both planes are vertical. For all of the measurement stations, the mean velocity and turbulent kinetic energy fields from the in-plane velocity components are determined (see section Evaluation and equations 8-11).
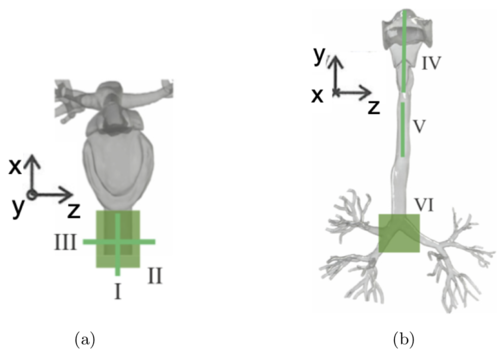
Description of experiment
The lung model was placed in an open liquid tank with a piston diaphragm pump attached to a linear actuator to achieve a quasi-stationary inspiratory flow. The stroke of the piston followed a cyclic triangular function with an adjustable falling constant slope and thus constant velocity to match different flow rates during inspiration. The employed working fluid is a mixture of water/glycerine (43:57 mass ratio, at ), which exactly matches the refractive index of the silicone model. Neutrally buoyant polyamide particles () were used as tracer particles (Stokes number Stk ≈ 0.002). Particle images were recorded using a CCD camera (pco.1600, PCO) with a resolution of 1600px x 1200px in double-frame mode. A total number of 688 image pairs were acquired for each measurement. Illumination was provided by a double-pulse Nd:YAG laser ( = 532nm, MinilitePIV, Continuum, 10 mJ per pulse). Images were evaluated by DaVis 8 (LaVision). The final grid size is 32 x 32px (planes I-III) and 16 x 16px (planes IV-VI), respectively, both with 50% overlap.
Boundary conditions
The mouth inlet and distal outlets of the lung model are open to the surrounding liquid. Thus liquid can enter and leave the model without any additional resistances or compliances. The local pressure at the mouth inlet corresponds to the barometric pressure plus the pressure of the water column above the model which is approximately 10cm. Accordingly, the pressure at the distal outlet increases by the additional water column which corresponds to the total model height of about 50cm. During inspiration, liquid is sucked into the model from the tank. The distance between tank's walls and the inlet of the model is about 15cm. The specific inlet conditions found in the experiment are presented in figure 7.
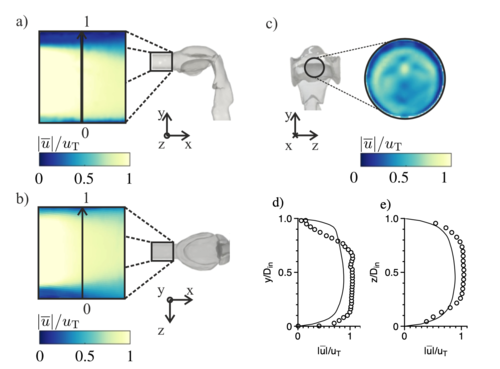
Measurement errors
For the experiments, the Reynolds similarity is calculated based on the properties of air at and atmospheric pressure, which results in a flow rate of = 31.75 L/min. This values has been used to program the linear actuator in order to provide the necessary main flow. At = 31.75 L/min, the experimental setup reaches its limit of the planned operation and a discrepancy between the targeted and actual occurring flow rate is observed. Thus, a maximum flowrate of = 28.56 L/min is achieved. This lowers the theoretical maximum Reynolds number from 4921 to 4286 but the flow still stays well within the turbulent regime. The reduction of the maximum Reynolds number occurs due to a leakage flow between the upper and lower part of the model. Consequently, the achieved flowrate within the main carina area is about 10% lower than in the upper part of the model. To account for this, we estimated the real flowrate based on the PIV data and recalculated the theoretical bulk velocity based on this estimate. This bulk velocity is then used as the correct scaling parameter for the results shown below. The measurement uncertainty of the displacement calculation for PIV is typically given as 0.1px (Adrian and Westerweel, 2011). With an adjusted mean displacement of around 8px this leads to a relative error of around 1.25% in the displacement calculation. Based on the proposed methods of Sciacchitano and Wieneke (2016) the statistical uncertainty of the derived mean velocity is around 0.05% and the statistical uncertainty of the turbulent kinetic energy estimation is around 3.
Measured data
The measured in-plane velocity and turbulent kinetic energy profiles at stations B-D and G,H,J (shown in figure 23 and 24), can be found in file PIV_profiles.xlsx.
Contributed by: P. Koullapisa, J. Muelab, O. Lehmkuhlc, F. Lizald, J. Jedelskyd, M. Jichad, T. Jankee, K. Bauere, M. Sommerfeldf, S. C. Kassinosa —
aDepartment of Mechanical and Manufacturing Engineering, University of Cyprus, Nicosia, Cyprus
bHeat and Mass Transfer Technological Centre, Universitat Politècnica de Catalunya, Terrassa, Spain
cBarcelona Supercomputing center, Barcelona, Spain
dFaculty of Mechanical Engineering, Brno University of Technology, Brno, Czech Republic
eInstitute of Mechanics and Fluid Dynamics, TU Bergakademie Freiberg, Freiberg, Germany
fInstitute Process Engineering, Otto von Guericke University, Halle (Saale), Germany
© copyright ERCOFTAC 2020